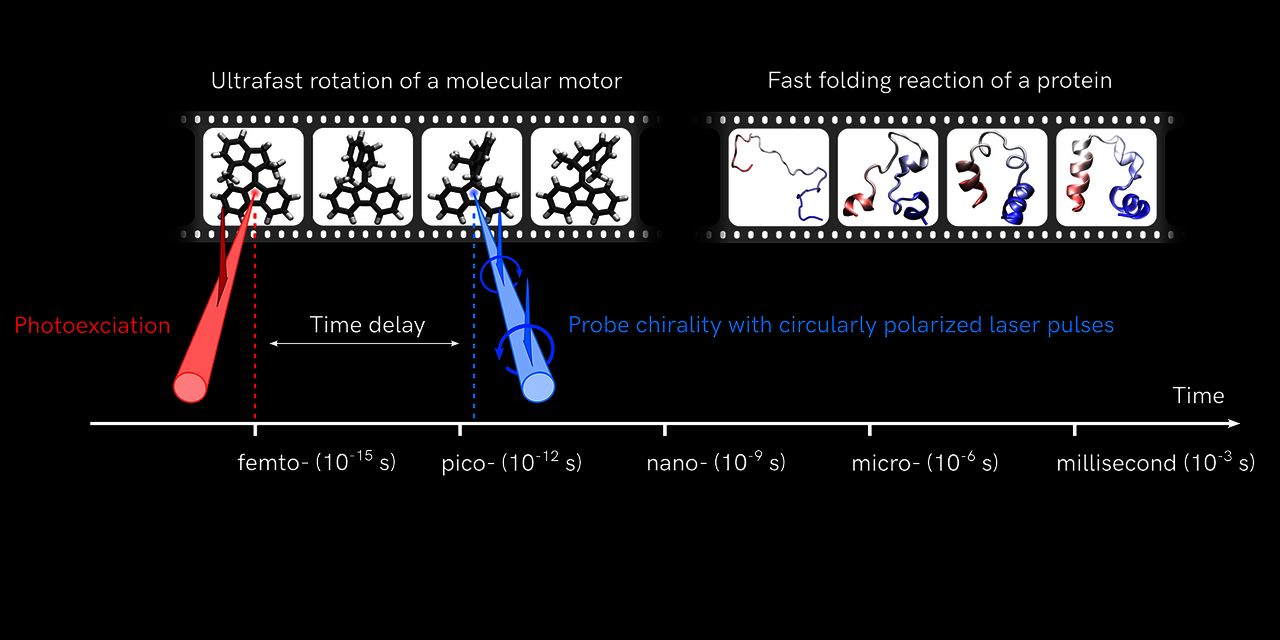
Research
1. Time-resolved chiral spectroscopy
The ability to follow changes in molecular structure during a chemical reaction or biological activity has been a dream of scientists for decades. However, the vast time and length scales covered by molecular motion turn this into a formidable experimental challenge: whilst the light-powered rotation of a synthetic molecular motor around a chemical bond takes place on the femto- to picosecond time scale, even the fastest protein folding reactions are orders of magnitude slower, taking place on the nano- to millisecond time scale. Moreover, resolving both local and global conformational motion on sub-millisecond time scales is particularly difficult in the native solution phase of most (bio-)chemical processes, where even the most established analytical techniques such as nuclear magnetic resonance and X-Ray scattering can reach limitations.
To address this challenge, we have developed a complementary experimental approach, based on detecting changes in the structural symmetry of molecules called chirality. We achieve this with a technique called circular dichroism (CD), which measures the absorption difference of left- and right-circularly polarized light in chiral molecules. In the (bio-)chemical sciences CD spectroscopy is routinely used to characterize the solution structures of proteins, DNA and chiral chemical compounds.
Our work takes CD spectroscopy to the time domain: we employ ultrafast laser technology to generate circularly polarized laser pulses to measure changes in CD with femtosecond time resolution. On this basis, we can follow the evolution of the molecule’s chiral structure in real-time, for example during a chemical reaction. Towards this goal, we have pioneered a new approach to time-resolved CD spectroscopy, where an ultra-sensitive broadband detection scheme enables extremely fast data acquisition speeds with exceptionally high CD measurement sensitivities. While this has already opened entirely new research avenues, we continue to develop novel (chiral) ultrafast spectroscopic tools in our laboratory.
2. Chiral photochemistry
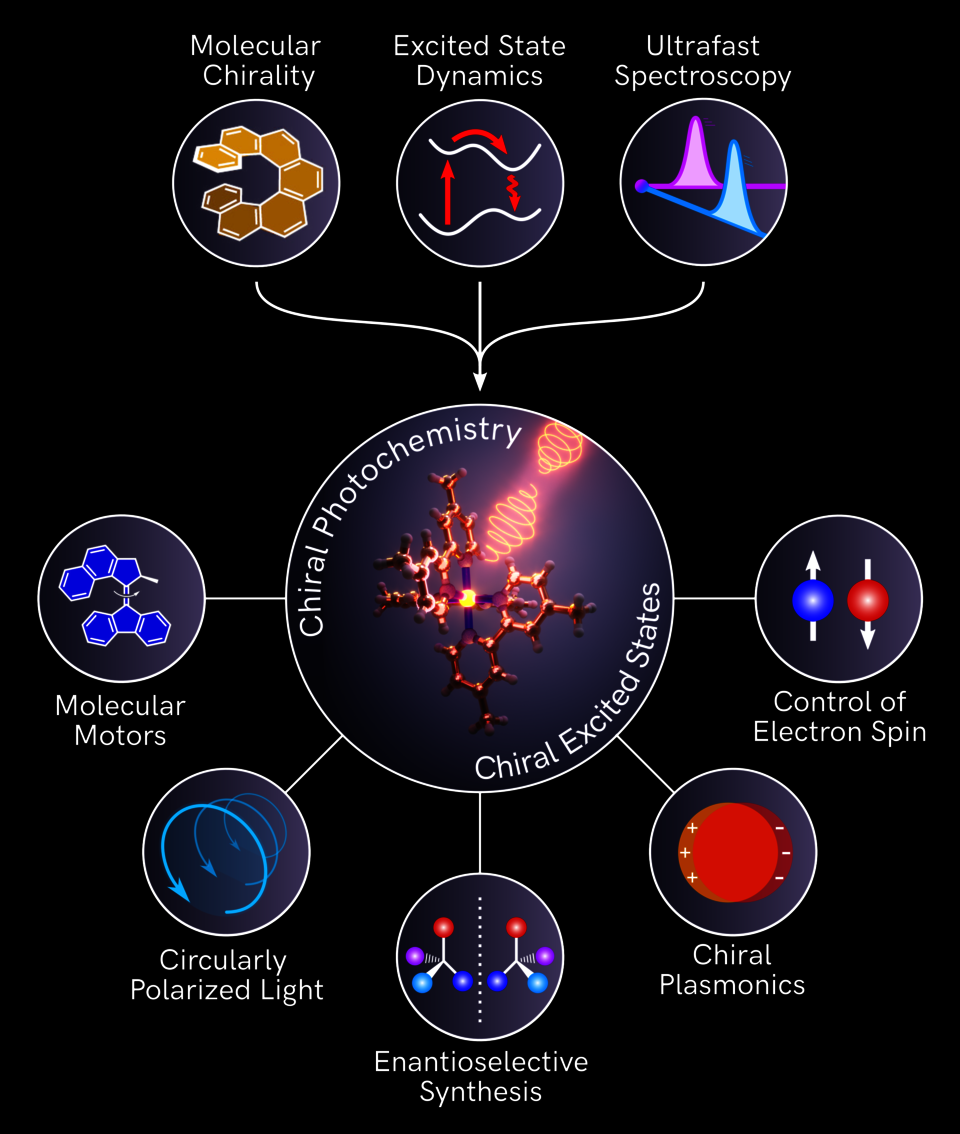
Chiral photochemistry focuses on the development of photoactive molecules and materials with chiral photoexcited states. These states are the basis for exciting photochemical applications, such as the unidirectional motion of molecular motors and the light-driven synthesis of enantiopure compounds. We investigate these phenomena with the help of ultrafast chiral spectroscopic tools (image by Malte Oppermann).
Chiral molecules exist in two forms, called enantiomers, which are mirror images of each other but non-superimposable. Even though enantiomers share most chemical and physical properties, they may differ greatly in their (bio-)chemical activities. Consequently, the identification and control of molecular chirality is essential for the design and synthesis of (bio-)molecular systems with bespoke functions, for example for the pharmaceutical, food and fragrance industries.
In this spirit, the incorporation of chiral structures into photoactive molecular systems has emerged as a powerful strategy to design novel photochemical functions: light-driven molecular motors for nanoscale synthetic machines, metallo-organic complexes for circularly polarized luminescence and enantioselective photocatalysis, organic helicenes for spintronic devices and chiral metal nanostructures for chiral sensing and enantioselective photochemistry. Through the chirality of their photoexcited states, these systems permit unprecedented levels of stereocontrol of mechanical work, energy transfer, electric charge carriers, and optical properties on the nanoscale. However, compared to these impressive synthetic advances, progress in developing key analytical tools has been slow. Indeed, resolving the chiral features of photoexcited states has remained a formidable experimental challenge and continues to limit the rational design and optimization of chiral photochemical materials.
With our approach to time-resolved circular dichroism (CD) it is now possible extract the CD spectrum of photoexcited states and follow their evolution on the time scale of the excited state dynamics. This is opening the path to directly resolve the coupling of the chiral molecular structure to the ensuing photochemical and -physical processes. In a joint effort with experts in chiral chemistry, we are applying our unique spectroscopic tools to understand and improve the stereocontrol of photochemical processes in photoactive chiral molecules and materials.
3. Ultrafast spectroscopy and photochemistry in the deep ultraviolet
Over the past decades, advances in laser technology have enabled ultrafast spectroscopic measurements across almost the entire electromagnetic spectrum, from the terahertz to the hard X-Ray. However, one spectral window has mostly remained elusive: the deep ultraviolet (UV) spanning 190-300 nm in wavelength. Closing this spectral gap is particularly attractive from an analytical perspective, as many sample classes contain characteristic UV chromophores which absorb below 300 nm: amino acids and peptide bonds in proteins, nucleobases in DNA, many organic ligands in chemical compounds, plasmonic transitions in several p-block metals, and large band-gap transitions in semiconductor materials.
As a first step towards ultrafast deep-UV spectroscopy, numerous nonlinear optical processes in crystalline media have been developed to generate broadband femtosecond laser pulses reaching down to about 250 nm. In this regime our group has acquired a strong expertise in the generation and application of broadband deep-UV laser pulses covering 250 – 400 nm for ultrafast spectroscopic techniques, including transient absorption, transient absorption anisotropy, 2-dimensional pump-probe spectroscopy and time-resolved circular dichroism. In our research, we apply these techniques to explore the photochemistry of coordination complexes, metal nanoparticles, and biological systems, such as DNA and proteins, via the electronic and structural dynamics encoded in their UV chromophores.
In this respect, one of our principal research goals is to overcome current limitations in ultrafast spectroscopy and gain broadband access to the entire deep-UV window. Towards this goal we develop novel optical setups and adopt advanced photonic technologies in collaboration with the Laboratory of Ultrafast Photonics at Heriot-Watt University in Edinburgh.
Our collaborators
Dr. Chris Brahms & Prof. John Travers (Heriot-Watt University, United Kingdom)
Dr. Jochen Brandt (Queen Mary University, United Kingdom)
Prof. Thomas Bürgi (Université de Genève, Switzerland)
Prof. Majed Chergui (École Polytechnique Fédérale de Lausanne, Switzerland)
Prof. Ben Feringa (University of Groningen, The Netherlands)
Prof. Peer Fischer (Universität Heidelberg, Germany)
Prof. Matthew Fuchter (Imperial College London, United Kingdom)
Dr. Jan Helbing (Universität Zürich, Switzerland)
Prof. Jérome Lacour (Université de Genève, Switzerland)
Dr. Jérémy Rouxel (Université de Rennes, France)
Prof. Josef Wachtveitl (Goethe Universität Frankfurt, Germany)
Dr. Francesco Zinna (Università di Pisa, Italy)